The end mill helix angle is one of the most critical geometric parameters that significantly impacts cutting performance, tool life, and machining quality. This spiral-shaped feature not only determines how effectively the tool cuts through various materials but also influences chip evacuation, heat dissipation, and overall cutting stability. Whether you’re working with aluminum, stainless steel, or hardened materials, understanding the proper helix angle selection can dramatically improve your machining results and tool longevity.
If you don’t want to read the article, you can also check out the comparison table of various end mill helix angles at the end.
What Defines an End Mill Helix Angle?
The helix angle end mill design is geometrically defined as the angle between the cutting edge tangent vector and the rotational axis of the tool. In simpler terms, it’s the angle of the spiral flutes wrapped around the tool body. This angle directly affects how the cutting edge engages with the workpiece material, determining the cutting forces generated during machining operations.
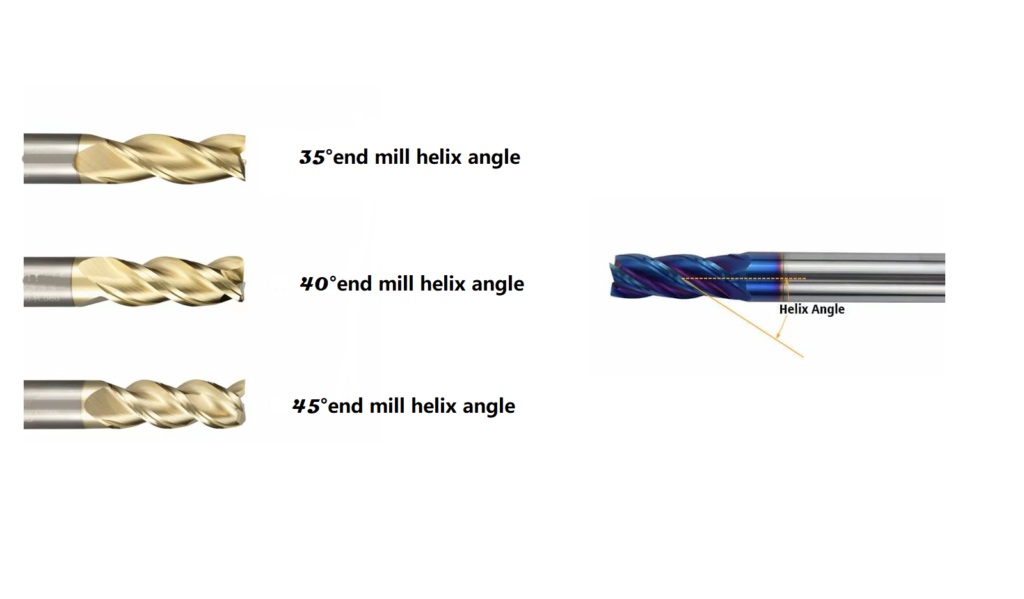
Helix Angle Formula and Mathematical Expression
For cylindrical end mills, the helix angle formula can be expressed through this fundamental equation:
tan(α) = r/T
Where:
- α represents the helix angle
- r is the radius of the end mill
- T is the lead (the axial distance required for one complete revolution of the helix)
In a more comprehensive mathematical context, the helix angle reflects the ratio between the tangential velocity component and the combined radial and axial velocity components:
tan(β) = V₍ₜ₎/√(V₍ᵣ₎² + V₍ᶻ₎²)
Understanding these relationships is essential when using a helix angle calculator to determine the optimal design parameters for specific applications.
Dynamic Variation in Complex Tools
In more complex rotary cutting tools like ball end mills and tapered tools, the helix angle may vary along different positions of the cutting edge. For example:
- In ball end mills, the helix angle gradually changes from the cylindrical portion to the ball-shaped tip
- In drill bits, the helix angle is typically largest at the outer edge (about 25°-32°) and decreases towards the center (as low as 6°)
- In tapered end mills, the helix angle must be carefully designed to maintain consistent cutting mechanics throughout the tool
Common End Mill Helix Angle Variations
Standard carbide end mills typically feature helix angles in three main categories:
- Standard helix (30°): Provides good balance between rigidity and cutting efficiency
- Medium helix (45°): Often referred to as a “power helix angle,” offering improved chip evacuation
- High helix end mill (60°): Specialized for challenging materials and high-performance applications
The recommended design range generally falls between 30° and 45°. Angles exceeding 45° may compromise tool rigidity, while angles below 30° can induce vibration and chatter during machining operations.
How Helix Angle Affects Machining Performance
Cutting Forces and Tool Geometry
A larger end mill helix angle effectively:
- Reduces radial cutting forces
- Improves the actual working rake angle
- Creates sharper cutting edges
- Distributes cutting load over a larger portion of the edge
This is particularly beneficial when selecting an end mill helix angle for aluminum and other soft materials, where minimizing built-up edge and ensuring smooth chip flow are critical.
Stability and Vibration Control
The helix angle enables gradual tool engagement with the workpiece, increasing the number of cutting edges in simultaneous contact. This characteristic:
- Minimizes impact loading
- Reduces vibration
- Provides smoother cutting action
- Improves surface finish quality
An end mill helix angle example demonstrating this advantage is seen in cylindrical end mills used for interrupted cutting operations, where higher helix angles distribute cutting forces more evenly, reducing tool wear.
Thermal Management and Tool Longevity
The helix angle significantly impacts heat generation and dissipation during machining:
- Higher helix angles extend the chip-tool contact path, promoting better heat diffusion
- This extended contact reduces heat concentration at a single point
- Improved heat distribution helps preserve the cutting edge integrity
- More efficient cooling allows for higher cutting speeds in appropriate applications
Material-Specific Helix Angle Selection
End Mill Helix Angle for Aluminum
When machining aluminum, selecting the proper helix angle is crucial:
- Recommended range: 40°-60°
- High helix end mill designs (around 45°-60°) are preferred
- Benefits include reduced heat generation, improved chip evacuation, and prevention of material adhesion
- The high helix angle enhances the sharpness of cutting edges, which is ideal for this soft material
- For aerospace-grade aluminum alloys (like 7075-T6), 50°-55° helix angles provide optimal performance
- When performing high-speed machining of aluminum, helix angles of 50°+ paired with appropriate coating technology can achieve exceptional results
End Mill Helix Angle for Stainless Steel
End mill helix angle for stainless steel presents unique challenges that require specific considerations:
- Recommended range: 35°-45°
- Medium to high helix angles work best
- The 45° “power helix” offers an excellent balance between cutting efficiency and heat management
- For tougher stainless steel grades, a 60° helix angle helps achieve better chip breaking and prevents work hardening
- Austenitic stainless steels (304, 316) benefit from helix angles in the 40°-45° range
- Martensitic and precipitation-hardened stainless steels may require lower angles (35°-40°) for improved edge strength
Helix Angles for Hard Materials
For hardened steels (HRC ≥ 50) and other difficult-to-machine materials:
- Recommended range: 30° or lower for maximum rigidity
- Variable helix end mill designs may be optimal
- Lower angles provide better edge support and resistance to chipping
- The increased tool strength compensates for the higher cutting forces required
- For tool steels and hardened mold components, 25°-30° angles provide the best balance of performance and tool life
- When machining titanium alloys, a moderate helix angle (35°-40°) helps manage the material’s poor thermal conductivity
Advanced Helix Designs
Variable Helix End Mill Technology
Variable helix end mill tools feature different helix angles along the same cutting tool, typically ranging from 30° to 45° in gradual variation. These specialized tools offer several advantages:
- Disruption of harmonic resonance patterns
- Significant reduction in chatter and vibration
- Improved stability during high-speed machining
- Enhanced surface finish quality
This advanced design is particularly beneficial when machining complex contours or when working with less rigid setups.
Variable Pitch Design Integration
Modern cutting tool design often combines variable helix angles with variable pitch spacing:
- Variable pitch disrupts the timing of tooth impacts
- When combined with variable helix angles, this creates a powerful anti-vibration system
- These tools excel in challenging applications like deep pocketing and thin-wall machining
- Industry testing has shown up to 80% reduction in harmonic chatter in certain applications
Directional Considerations
The direction of the helix angle also matters:
- Right-hand helix angles facilitate upward chip evacuation
- Left-hand helix angles direct chips downward
- Selection should complement the spindle rotation direction of the machine tool
- In some materials, the direction can influence the quality of the machined edge
- For horizontal machining centers, the helix direction significantly impacts chip control and evacuation
Using a Helix Angle Calculator for Optimal Selection
When precision is critical, utilizing a helix angle calculator can help determine the ideal angle for specific applications. Factors to consider include:
- Material properties (hardness, thermal conductivity)
- Machine rigidity and power
- Desired surface finish
- Chip control requirements
- Tool life expectations
Online calculators often use the helix angle formula mentioned earlier, allowing machinists to input their specific parameters for customized recommendations.
Practical Calculation Example
To know how a helix angle calculator works:
For a 12mm diameter end mill with a lead (T) of 40mm:
- r = 6mm (radius)
- T = 40mm (lead)
- tan(α) = 6/40 = 0.15
- α = tan⁻¹(0.15) ≈ 8.53°
However, this angle would be too small for most applications. By adjusting the lead to 10mm:
- tan(α) = 6/10 = 0.6
- α = tan⁻¹(0.6) ≈ 31°
This demonstrates how tool designers manipulate the lead value to achieve desired helix angles for specific applications.
Balancing Tool Life and Machining Precision
Finding the optimal end mill helix angle involves balancing several competing factors:
- Tool life: Helix angles up to 40° generally improve tool life by distributing cutting loads, but angles exceeding this threshold may reduce rigidity
- Machining precision: Moderate helix angles (30°-40°) provide the best balance between vertical tolerance and flatness
- Material deformation: When machining thin-walled components, smaller helix angles reduce axial forces that could cause deformation
- Power consumption: Higher helix angles generally require less power, potentially allowing for increased cutting parameters
Industry Applications and Case Studies
Aerospace Component Manufacturing
In aerospace machining:
- High helix end mill tools (45°-60°) are preferred for aluminum structural components
- Variable helix end mill designs are essential for titanium frame components to manage vibration
- For Inconel and other nickel-based superalloys, specialized helix angles of 35°-40° combined with appropriate coatings yield optimal results
Die and Mold Production
For mold-making applications:
- Medium helix angles (35°-40°) provide the best balance for semi-finishing operations
- Lower helix angles (25°-30°) excel in hardened steel finishing cuts where surface finish is critical
- For deep cavity machining, specialized tools with progressively increasing helix angles maintain wall quality
End Mill Helix Angle Example Applications
Here are some end mill helix angle example scenarios to illustrate practical application:
- Aluminum Electronic Housing
- Material: 6061-T6 Aluminum
- Tool: 12mm diameter, 3-flute, 50° helix angle
- Result: Excellent surface finish with 25% higher feed rates than standard helix tools
- Stainless Steel Medical Components
- Material: 316L Stainless Steel
- Tool: 8mm diameter, 4-flute, 45° helix angle
- Result: Improved chip control and reduced work hardening
- Hardened Tool Steel Die Components
- Material: D2 Tool Steel (60 HRC)
- Tool: 6mm diameter, 4-flute, 30° helix angle
- Result: Enhanced edge durability and consistent dimensional accuracy
Abschluss
The end mill helix angle represents a fundamental design parameter that directly influences machining performance. By understanding the geometric principles and practical applications of different helix angles, machinists can make informed decisions to optimize cutting operations across various materials. Whether working with aluminum’s tendency to adhere to cutting edges or stainless steel’s work-hardening properties, selecting the appropriate helix angle—or implementing variable helix designs—can dramatically improve productivity and part quality.
What is the best helix angle for aluminum machining?
For aluminum machining, higher helix angles between 40° and 60° are generally optimal. These angles provide sharper cutting action, reduce heat generation, and improve chip evacuation, which helps prevent the aluminum from adhering to the tool.
How does helix angle affect tool life?
Helix angle affects tool life by influencing cutting forces and heat distribution. Moderate angles (35°-45°) typically maximize tool life by balancing cutting efficiency and structural rigidity. Angles that are too high may weaken the cutting edge, while angles that are too low can increase vibration and friction.
What’s the difference between variable helix end mills and standard end mills?
Variable helix end mills feature different helix angles across different flutes, while standard end mills maintain consistent helix angles. Variable designs disrupt harmonic vibrations, reduce chatter, and improve stability, particularly beneficial for challenging materials or less rigid setups.
Can I calculate the optimal helix angle for my specific application?
Yes, you can use a helix angle calculator that incorporates the helix angle formula (tan(α) = r/T) along with material properties, cutting parameters, and machine capabilities to determine the optimal angle for your specific application.
When should I choose a high helix end mill versus a standard helix?
Choose a high helix end mill (45°-60°) when machining soft materials like aluminum, when chip evacuation is critical, or when surface finish quality is paramount. Standard helix tools (30°) are better suited for harder materials where edge strength and rigidity are more important than chip flow.
How do coatings interact with helix angle selection?
Coating technology works synergistically with helix angle design. Higher helix angles often benefit from low-friction coatings like TiAlN or AlTiN to enhance chip flow, while lower helix angles may use harder coatings like TiCN to reinforce edge strength in tough applications.
Are there situations where unusual helix angles are recommended?
Yes, in specialized applications like composite material machining, extra-high helix angles (65°+) may be used to minimize delamination. Conversely, micro-machining operations may utilize very low helix angles (15°-20°) to maximize tool rigidity at small diameters.
End Mill Helix Angle Comparison Table
Helix Angle | Typical Range | Ideal Applications | Materials | Advantages | Disadvantages | Schneidkräfte | Chip Evacuation |
---|---|---|---|---|---|---|---|
Low Angle | 15°-30° | Heavy roughing, Hard materials, Slot milling | Tool steel, Hardened steel (>50 HRC), Cast iron |
• Higher tool rigidity • Better edge strength • Improved stability in slots • Higher resistance to chipping |
• Increased vibration potential • Less efficient chip evacuation • Higher cutting temperatures • More aggressive entry into workpiece |
• Higher radial forces • Lower axial forces • Higher power consumption | Slower chip removal Less efficient in deep pockets |
Medium Angle | 30°-45° | General purpose milling, Semi-finishing, Most standard applications | Carbon steel, Alloy steel, Pre-hardened steel (30-50 HRC), Stainless steel |
• Good balance of rigidity and cutting efficiency • Versatile across materials • Moderate heat generation • Balanced chip flow |
• Not optimized for extreme conditions • Average performance in most categories |
• Balanced radial and axial forces • Moderate power requirements | Medium efficiency Good for most applications |
High Angle | 45°-60° | High-speed machining, Finishing operations, Thin-wall components | Aluminum, Copper, Brass, Non-ferrous alloys, Plastics |
• Sharper cutting action • Superior chip evacuation • Reduced cutting forces • Better surface finish • Less heat concentration |
• Reduced tool rigidity • Potential deflection in heavy cuts • Less suitable for interrupted cutting |
• Lower radial forces • Higher axial forces • Lower power consumption | Very efficient Excellent for deep pockets Prevents chip recutting |
Extra-High Angle | >60° | Ultra-high-speed machining, Specialized finishing, Composite materials | Soft aluminum, Plastics, Carbon fiber composites, Graphite |
• Extremely efficient chip evacuation • Minimal cutting pressure • Excellent for delicate materials • Best for preventing delamination |
• Poor rigidity • Prone to deflection • Limited depth of cut capability • Susceptible to premature wear |
• Minimal radial forces • Maximum axial forces • Lowest power consumption | Extremely efficient Ideal for deep cavity machining |
Variable Helix | Mixed (typically 35°-50° with 3°-8° variations) | Vibration-prone setups, Thin walls, Deep pockets, Long overhang conditions | All materials, particularly effective in difficult-to-machine alloys |
• Excellent vibration suppression • Reduced harmonics and chatter • Improved surface finish in challenging conditions • Enhanced tool life in vibration-prone applications |
• Higher manufacturing cost • More complex resharpening • Application-specific performance • Less standardized |
• Distributed force patterns • Disrupted harmonics • Optimized stability | Variable efficiency based on design Generally excellent in difficult conditions |